While working with Amaldi to establish the optimal irradiation conditions of a silver sample, he realized that the sample acquired much more activity if placed on a wooden table instead of marble. The results were reported to Fermi who guessed a possible explanation and started to irradiate the silver cylinder by placing a paraffin layer between source and sample.
The silver resulted much more radioactive than when directly irradiated. For Fermi everything was clear:
a hydrogenated substance such as paraffin, and in part the wood, was much more effective in slowing down the neutrons and therefore neutrons had a greater probability to be captured by nuclei and cause higher activity.
It was October 20, 1934.
The slowing down effect was also tested on materials that, until then, had not been activated. The discovery was published on October 22 with a
Letter, signed by Fermi and his collaborators, to La Ricerca Scientifica, the National Research Council journal. The Director of the Institute of Physics, Orso Mario Corbino, suggested to patent the process. Four days later, at the Ministry of Corporations - Intellectual Property Office, Fermi filed the
application for the patent on:
"Method to increase the performance in the process of producing artificial radioactivity by means of neutron bombardment". The document was signed by all his collaborators.
The patent, number 324458, was registered on February 2, 1935. After Italy, it was then extended to other countries resulting very useful to the inventors when the neutron slowing down process began to be used in nuclear fission and for the production of new radioactive isotopes.
In 1938, Fermi was awarded the Nobel Prize
"for the demonstrations of the existence of new radioactive elements produced by neutron irradiation, and for his related discovery of nuclear reactions brought about by slow neutrons".
From November 1, 1934 up to June 28, 1936 Pontecorvo was appointed
assistant at the Institute of Physics of the Royal University of Rome. During this period he conducted systematic studies on slow neutrons to confirm the effect of hydrogenated substances and check the absorption power of slow neutrons in various materials. On 7 November a
second Letter was sent to La Ricerca Scientifica with the results of this work. Only three authors had signed it: Fermi, Pontecorvo and Rasetti.
The study of the properties of slow neutrons was continued by Pontecorvo who published a
paper "on the properties of slow neutrons" (Nuovo Cimento, April 1935).
Before the end of his contract Pontecorvo won a scholarship from the Ministry of National Education. Funded by the award he decided to
resign from his position and went to work in Paris to the Institut du Radium and then to the Collège de France. Thanks to a
letter of introduction by Enrico Fermi he contacted Frédéric and Irène Joliot-Curie, respectively son-in-law and daughter of Pierre and Marie Curie. In France he successfully continued his studies on slow neutrons showing that there was an
inhomogeneity of the γ radiation emitted in neutron capture.
As a consequence of the studies of the γ radiation inhomogeneity and from its interpretation in terms of neutron capture in
different but very close nuclear levels, Pontecorvo started to be interested to the
nuclear isomerism and to the idea that could exist β-stable isomers, i.e. non-emitting β radiation. In 1938 he produced together with Maurice Dodé the first
metastable isomer of Cadmium under the action of fast neutrons and in 1939 he discovered the phenomenon called the
nuclear phosphorescence for which earned the Curie-Carnegie Award. With André Lazard, who designed the Van de Graaff generator for the Ivry accelerator, obtained the first
stable β isomer of Indium (115*In) by irradiating stable nuclei with a continuous high-energy X-ray spectrum (~ 3 MeV).
In 1940, to escape the German invasion of Paris, Pontecorvo leaved with his family for the United States and settled in Tulsa, Oklahoma, where he worked for about two years for the Well Surveys Inc.,
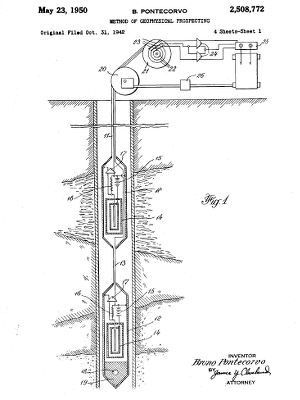
an American private company specialized in oil prospecting. Here Pontecorvo developed an original geophysical survey method for oil wells, the so-called
neutron well logging. The system, based on the neutron slowing down process, used a neutron source (radium-beryllium) and an ionization chamber, carefully shielded by the radiation coming directly from the source. The information on the nature of the geological formations in the different ground layers was obtained to by measuring the returning radiation (particularly gamma and neutrons) emitted by the formations as a result of the primary irradiation. This method, immediately adopted on a large scale in the oil fields, is still used. Bruno did not patent it, demonstrating once more how his thoughts were focused on pure research only.
Those years were, for Bruno, years of great satisfaction, quiet life, constant contacts, even if not frequent, with Fermi and Segrè and of assiduous epistolary relationships, in particular with his friend Giancarlo Wick. But suddenly any contact was lost.
It was 1942 and in the USA it was starting in great secret the Manhattan Project and the construction of the Los Alamos Laboratory in New Mexico. The race to develop the atomic bomb had began also in England. By the spring of 1942, British scientists had made significant progress on determining the feasibility of an atomic bomb. In Cambridge, a team, coalesced around Hans von Halban and Lew Kowarski who were escaped from France at the time of the German invasion, had shown in experiments with uranium oxide and heavy water (the 40 gallons brought from France) that a sufficient increase in neutron and fission activity could be achieved and a self-sustaining chain reaction could be obtained.
The
MAUD Report of the Scientific Committee formed during the Second World War to establish whether the atomic bomb was practical, opened with the following statement:
"We should like to emphasize at the beginning of this report that we entered the project with more skepticism than belief, though we felt it was a matter which had to be investigated. As we proceeded we became more and more convinced that release of atomic energy on a large scale is possible and that conditions can be chosen which would make it a very powerful weapon of war. We have now reached the conclusion that it will be possible to make an effective uranium bomb which, containing some 25 lb of active material, would be equivalent as regards destructive effect to 1,800 tons of TNT and would also release large quantities of radioactive substances which would make places near to where the bomb exploded dangerous to human life for a long period."
In response of this report a nuclear weapons project, officially named
Tube Alloys, was created.
To avoid the results of the British nuclear research could fall into German hands, the British Government, in agreement with the Canadian government, decided to transfer the Tube Alloys project from the Cambridge's Cavendish Laboratory to Canada. So the Halban's team and the equipment of the Laboratory moved to Montréal. The first group of scientists arrived from England in December 1942. Initially the research was conducted in an old residence belonging to the McGill University, at 3470 Simpson Street, but a few months later the laboratory was transferred to a new, larger University building. The project was funded by the British Department of Scientific and Industrial Research and by the Canadian National Research Council.
Hans von Halban was
the first director of the laboratory. George Placzek was the head of the Theoretical Division, Pierre Auger of the Experimental Division.
Bruno was contacted by old friends and colleagues of Paris, Pierre Auger, Bertrand Goldschmidt and of course von Halban, all involved in the nuclear program for the construction of a pilot plant for the production of plutonium.
On February 7, 1943 Pontecorvo and his family moved to Montreal and settled in an apartment about 2 miles from the city center, at the extreme south of Mount Royal.
The staff of the laboratory grew quickly, arriving in a short time to more than three hundred people among scientists, technicians and engineers. Scientists immediately began investigating the penetration of neutrons through the different kinds of materials that might be used for the reactor core, such as heavy water, graphite, and combinations of these with uranium and other materials.
In those years Bruno visited Fermi in Chicago several times. He wanted to discuss with him the results on the absorption of neutrons in fissionable materials such as U-235 and Pu-239. Two years earlier, on December 2, 1942, Fermi had turned on the first self-sustained nuclear reactor in history, the so called Chicago Pile-1. The pile consisted of a lattice structure of pure graphite bricks embedded with uranium oxide beads and pure uranium. Cadmium control rods were used to absorb excess free neutrons and prevent a chain reaction out of control.
Thanks to the excellent results obtained by the scientists involved in the Anglo-Canadian nuclear research program and despite initial discouragement due to the lack of collaboration from American scientists and the poor administration of the laboratory by the director von Halban, on April 13, 1944, it was decided to proceed with the design and construction of a heavy water moderated nuclear reactor. The English Physicist John Cockcroft was the new director of the laboratory in place of von Halban.
The National Research Council of Canada selected Chalk River, Ontario as the site for one of the world's first heavy water nuclear reactors. The construction of the reactor, known as
the Zero Energy Experimental Pile (ZEEP) 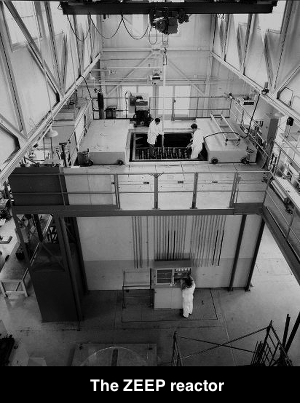
, began in August 1944. The ZEEP reactor was designed to use natural uranium, which allowed physicists to bypass the complex and expensive process of uranium enrichement. It went critical at 3:45pm on September 5, 1945, three days after representatives from the Japanese government signed the Japanese Instrument of Surrender which effectively ended the Second World War.
The ZEEP was a low-power prototype reactor. It was very useful for lattice studies and to understand physics and problems of heavy water moderated nuclear reactions. It provided important data for the design of the powerful
National Research Experimental (NRX) reactor, aimed at producing plutonium from natural uranium and U-233 from thorium.
Pontecorvo played a fundamental role in the design of the NRX reactor being a member of the
Lattice group that defined the characteristics of the reactor (size and geometrical shape) for the required energy output. He studied the neutrons flux in uranium/heavy water assembly and was responsible for the design of the shielding system in connection with heat and neutron diffusion.
In those years Pontecorvo received
job offers from the most prestigious American universities, but he renounced such opportunities to follow the work on the NRX reactor. Between 1945 and 1946 he developed a boron trifloride detector (BF3) to monitor the neutron flux in the start-up phase. NRX went into operation on July 22, 1947 with an initial power of 20MW and Bruno was one of the four physicists allowed to enter the Control Room. It was a very powerful and versatile machine and it was used not only for research purposes but also for the production of medical radioisotopes such as Iodine-131, Phosphorus-32, Carbon-14 and Cobalt-60.
In those years Bruno also developed an intensive research program in the field of elementary particles. He was particularly interested in a particle called the "neutrino", considered extremely elusive and impossible to be detected.
Soon after the famous 1934 Fermi paper on the theory of
β-decay, Bethe and Peierls estimated the interaction cross section of neutrinos with nuclei extremely small (σ < 10
-44 cm
2). For many years, the neutrino was considered as an
undetectable particle. Wolfgang Pauli, who first suggested the existence of the neutrino in 1930, even wrote
"I have done something very bad today by proposing a particle that cannot be detected; it is something no theorist should ever do."
Pontecorvo was the first who challenged this opinion and in 1946 he proposed a
radiochemical method for the neutrino detection. The method was based on the observation of the decay of the daughter nucleus produced in the reaction
ν + (A,Z) → e- + (A, Z+1). He selected the reaction:
νe + 37Cl → e- + 37Ar
which occurs in tetrachlorethylene (C
2Cl
4) when irradiated by ν
e. Such reaction was very promising for many reasons: C
2Cl
4 is a cheap and non-flammable liquid,
37Ar nuclei are unstable with a convenient half-life (34.8 days), a few atoms of the rare gas, produced during the exposition time, can be extracted from a large detector.
The Pontecorvo Cl-Ar method was used by Raimond Davis Jr. in his
pioneering experiment on the detection of solar neutrinos for which he was awarded the Nobel Prize in 2002.
Pontecorvo studied in depth also another elementary particle: the muon. After the Conversi, Pancini, and Piccioni
experiment and the
theoretical analysis provided by Fermi and Teller, in collaboration with Weisskopf, according to which the capture of a mesotron by a nucleus was 10
12 times less probable than predicted, it was clear that the mesotron produced in cosmic rays was not the strongly interacting Yukawa particle (today pion). Pontecorvo wrote his
ideas about mesotron to his friend Giancarlo Wick. He wanted to know what Wick was thinking about his hypothesis that the mesotron could be a
"sort of isomer" of the electron and suggested to search for its decay in electron plus photon or electron plus a spin-zero particle. After reading
Fermi and Teller's article on the capture of negative mesotrons in the matter, Pontecorvo published the article entitled
"Nuclear capture of mesons and the meson decay" on the Physical Review.
In the paper, Pontecorvo claimed that
"the probability of capture of a bound negative meson is of the order of the probability of ordinary K-capture processes, when allowance is made for the difference in the disintegration energy and the difference in the volumes of the K-shell and of the meson orbit" and suggested
"the possibility of a fundamental analogy between β--processes and processes of emission and absorption of charged mesons".
It was the key intuition at the base of the
"Universality of weak interactions".
Unfortunately the paper had not a large echo and 2 years later Giampietro Puppi introduced the concept of
"Universal weak interaction" without any reference to the previous Pontecorvo ideas.
With Ted Hincks, Bruno started accurate
experimental measures on cosmic rays from which he was convinced that the observed electrons came from the decay of muons in flight and developed a new technique of
proportional counters, based on very large amplification in the gas.
Bruno remained in Canada until January 1949 when, naturalized British citizen, he moved to England to work at the Atomic Energy Research Establishment in Harwell, the nuclear research center directed by John Cockcroft. In September 1949 he partecipated to the Basel and Como International Conference on
"Nuclear physics, quantum electrodynamics and cosmic rays", organized jointly by the Italian and the Swiss Physics Society where he presented a work on
"Recent developments of the proportional counter technique", published in
Helvetica Physica Acta.
Two months later, he went to Edinburgh at the
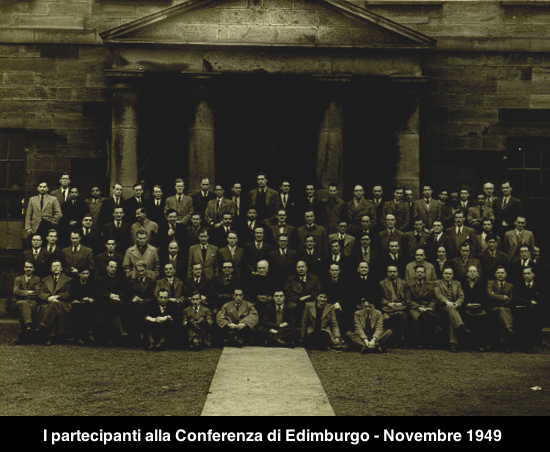
International Conference on Elementary Particles where he presented a talk
"On the decay products of the μ-meson".
Bruno's stay in Harwell lasted only a bit more than a year. High levels of the Military Intelligence MI5, the UK Security Service, were investigating him and his family for alleged communist beliefs. On May 10, 1950 Roger Hollis, the Director General of MI5, decided it was a
security risk to leave Pontecorvo in Harwell and welcomed the possibility to move him to the University of Liverpool. There, Professor Herbert Skinner was enthusiastic that Bruno might join his Department and offered him the chair of physics. Skinner thought that the scientific possibilities at the Liverpool University, where a large synchro-cyclotron was being built, would have exited him.
When Pontecorvo visited Liverpool was not particularly impressed nevertheless on July 24, 1950
he wrote his definitive decision to the vice-chancellor James Mountford. He accepted the professorship and would have moved there in autumn, after a summer vacation in Italy.
On July 25 he left Abingdon, near Harwell, with his family and his sister Anna, a teacher living in London. At Dover they took the overnight ferry to Dunkirk. Bruno drove through France and Switzerland, arriving at Menaggio, near Lake Como, on 31 July. There they camped until the 6th of August then went for a couple of days to the Dolomites, before moving to Rome. The Pontecorvo family spent most of the time at the sea near Rome, between Ladispoli and Circeo, and only towards the end of August moved to Rome, after the thirty-seventh birthday of Bruno.
On August 29, Bruno booked 5 round-trip tickets for Stockholm at an agency of the Scandinavian Airlines. He paid the tickets 600 dollars in cash the next day, before embarking for the Swedish capital. The Pontecorvos never visited Marianne's mother but abruptly disappeared behind the iron curtain. For five long years no one knew anything about Pontecorvo and his family. Many years later, during a visit in Italy, Bruno told to the writer Miriam Mafai of his clandestine trip closed in the trunk of a car.
Bruno went to work in Dubna, a town about 150 km north of Moscow, at the
Institute for Nuclear Problems of the Academy of Sciences, where the largest accelerator in the world had just come into operation: a synchro-cyclotron that could accelerate protons up to 480 MeV as well as deuterons and α particles.
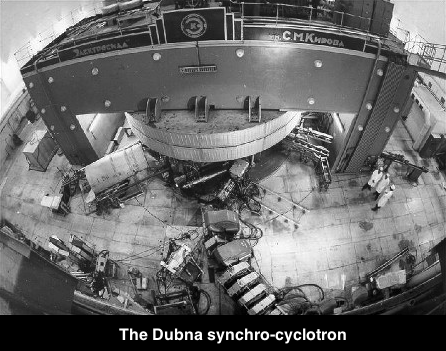
It was a great opportunity for the brilliant experimental physicist like he was.
From the accelerator it was possible extracting up to 14 beams (polarized and not) of different types of particles such as protons, neutrons, pions, muons and γ (from the π
o decay).
Bruno immediately started to study the fundamental characteristics of these beams, such as intensity, energy and angular distribution.
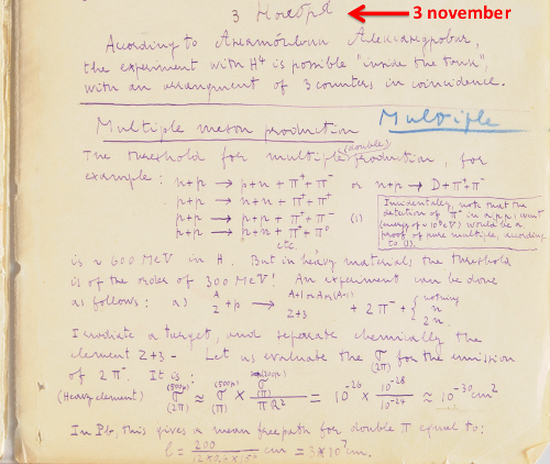
He was particularly interested in neutron beams and in the possibility of using them in experiments on pion production in n-p collisions. The
first page of his Logbook, dated November 1 (1950), begins infact with the study of the neutron beam obtained from the cyclotron. Pontecorvo proposed several experiments to study the multiple production of mesons (charged and neutral pions) in nucleon-nucleon collisions and meson decay. His interest in the production of pions by neutron beams was because until then many experiments had been carried on essentially by using proton beams only.
All the experiments conducted between 1951 and 1955 confirmed that proton and neutron, different particles from the point of view of electromagnetic interaction, could be considered the same particle in two different states of a new quantum number called
isotopic spin, as concerns strong interaction.
The scientific interest of Pontecorvo was not confined to experiments of scattering of nucleons and
π mesons on nuclei, but he deeply studied also the weak interactions and the so-called
strange particles discovered only a few years earlier by George Rochester and Clifford Butler in a
cosmic rays experiment. The characteristic of these particles is that they are produced with high probability typical of strong interactions but decay with relatively long lifetimes (10
-8-10
-10 sec). As Pontecorvo wrote in his logbook on
page 8,
"the contradiction between the existence of a strongly interacting particle and its long lifetime ... is resolved if the strong interacting particle is produced in pair". It is the hypothesis of
associated production and it is dated November 1950, two years before the
famous article by Abraham Pais on The Physical Review.
To verify his idea, a few years later Bruno proposed an experiment, at the Dubna accelerator, to check if it was possible to produce single Λ0 hyperons in strong interactions between protons and nucleons, since the energy of the accelerator was not enough to produce them in pair with the K mesons. The
results of the experiment confirmed his hypothesis.
In recognition of his research in 1953 he was awarded the prestigious Stalin Prize.
In 1956 Pontecorvo became the head of the experimental physics division of the Laboratory of Nuclear Problems whose Director, at the time, was Venedikt Dzhelepov.
In those years he continued his studies on the fundamental properties of the muon and on the nature of the particle emitted in the muon-nucleon interaction by using a diffusion cloud chamber filled with
3He (
reaction μ- + 3He → 3H + ν).
He revealed his deep scientific insight in the debates on the existence of different types of neutrino, where he provided a fundamental and decisive contribution. It was well known the enthusiasm with which he discussed the methods to experimentally demonstrate that
electron and muon neutrinos are different particles.
Impressed by the
K0⇄anti-K0 oscillations suggested by Gell-Mann and Pais (1955), in 1957 he published in the Russian Journal of Experimental and Theoretical Physics an
article where he treated the question
"whether there exist other 'mixed' neutral particles (not necessarily 'elementary') besides the K0 meson, which differ from their antiparticles and for which the particle→antiparticle transitions are not strictly forbidden." After considerations on baryon and fermion number conservation, he concluded that mixed systems could be the
mesonium, defined as the bound system (μ
+e
-), and the
antimesonium (μ
-e
+) and that mesonium ⇄ antimesonium transitions were possible. Furthermore, about the neutrino, he wrote that
"if the two-component neutrino theory should turn out to be incorrect ... and if the conservation low of neutrino charge would not apply not applied, then in principle the neutrino→antineutrino transitions could take place in vacuo."
In 1957-1958 only a two-component neutrino was known: a left-handed ν
L component and a right-handed ν̄
R one. Pontecorvo assumed that transitions ν̄
R → ν
R and ν
L → ν̄
L were possible. Thus not only the lepton number was not conserved but it existed, in addition to the standard right-handed antineutrino and left-handed neutrino, also a right-handed neutrino (ν
R) and a left-handed antineutrino (ν̄
L), both sterile from the weak interaction point of view. In 1967 Pontecorvo wrote a
second article on the neutrino oscillations. At that time the K
0⇄anti-K
0 oscillations had already been observed and it was proved the
existence of two types of neutrino: ν
μ e ν
e.
In the paper Pontecorvo also discussed the oscillations ν
μ ⇄ ν
e and observed that:
"If the oscillation length is large (> 10 km) it will be impossible to observe the transitions ν ⇄ ν̄ and νμ ⇄ νe in neutrino beams from reactors or accelerators. However, significant astrophysical effects may be possible. From the point of view of detection possibilities, an ideal object is the sun. If the oscillation length is much smaller than the radius of the solar region which effectively produces neutrinos (e.g. one tenth of the solar radius R☉ or 105 km for neutrinos from B8) .... it will be impossible to detect directly oscillations of the solar neutrinos, owing to a smearing out of the effect. The only effect at the surface of the earth would consist in the fact that the flow of observable solar neutrinos would be half as large as the total flux of solar neutrinos".
At the time of this article, Raymond Davis Jr. was preparing his famous solar neutrino experiment in the Homestake's gold mine in South Dakota. The
first results of that experiment showed that the detected solar neutrinos flux was smaller than the expectation from the Standard Solar Model. The
data collected between 1970 and 1994 (2.56 ± 0.16 SNU) confirmed a flux equal to about 1/3 of that provided by the theoretical model (~ 7 SNU).
Among the various possible astrophysical (and particle physics) explanations of this problem, the Pontecorvo
neutrino oscillations hypothesis proved to be the most natural one and it was soon accepted.
The existence of this phenomenon has been verified in several experiments such as
GALLEX,
SAGE,
GNO,
SNO,
Super-Kamiokande,
BOREXINO ...
After the Nobel Prize awarded in 2002 to Raymond Davis Jr. and Masatoshi Koshiba
"for their pioneering contributions to astrophysics and in particular for the detection of cosmic neutrinos", in 2015 the scientists Takaaki Kajita (Super-Kamiokande) and Arthur Bruce McDonald (SNO) received the same high recognition
"for the discovery of neutrino oscillations, which shows that neutrinos have mass".
The discovery of the neutrino oscillations was the triumph of the extraordinary intuition that Bruno Pontecorvo had in a period when the hypothesis of massless neutrinos was the most accredited. According to Samoil Bilenky, close collaborator and great friend of Pontecorvo
"the history of neutrino oscillations is an illustration of the importance of analogy in physics. It is also an illustration of the importance of new courageous ideas, not always in agreement with the general opinion".